Titled The Nernst Effect in Corbino Geometry, Prof. Kavokin’s latest research appeared recently in Proceedings of the National Academy of Sciences of the United States of America (PNAS). Alexey Kavokin, Chair Professor of Physics at Westlake University, is the first author, and is joined by correspondent author Boris Altshuler from Colombia University.
Kavokin and collaborators found that a Corbino disk geometry offers a precious opportunity for the observation of the specific Nernst effect having a purely thermodynamic nature. They predict, that, with an applied temperature difference between the outer and inner edges of the disk and a magnetic field perpendicular to the plane of the disk, there will be strong oscillations of the induced magnetic field in the center of the disk as a function of the external field.
The inspiration kicked in when Professor Boris Altshuler visited Westlake University in 2019. By the end of the three-week visit, Kavokin and Altshuler formed the concept and model. “In the following weeks, we completed the entire calculations, with only pens and paper. It is beautiful,” said Kavokin.
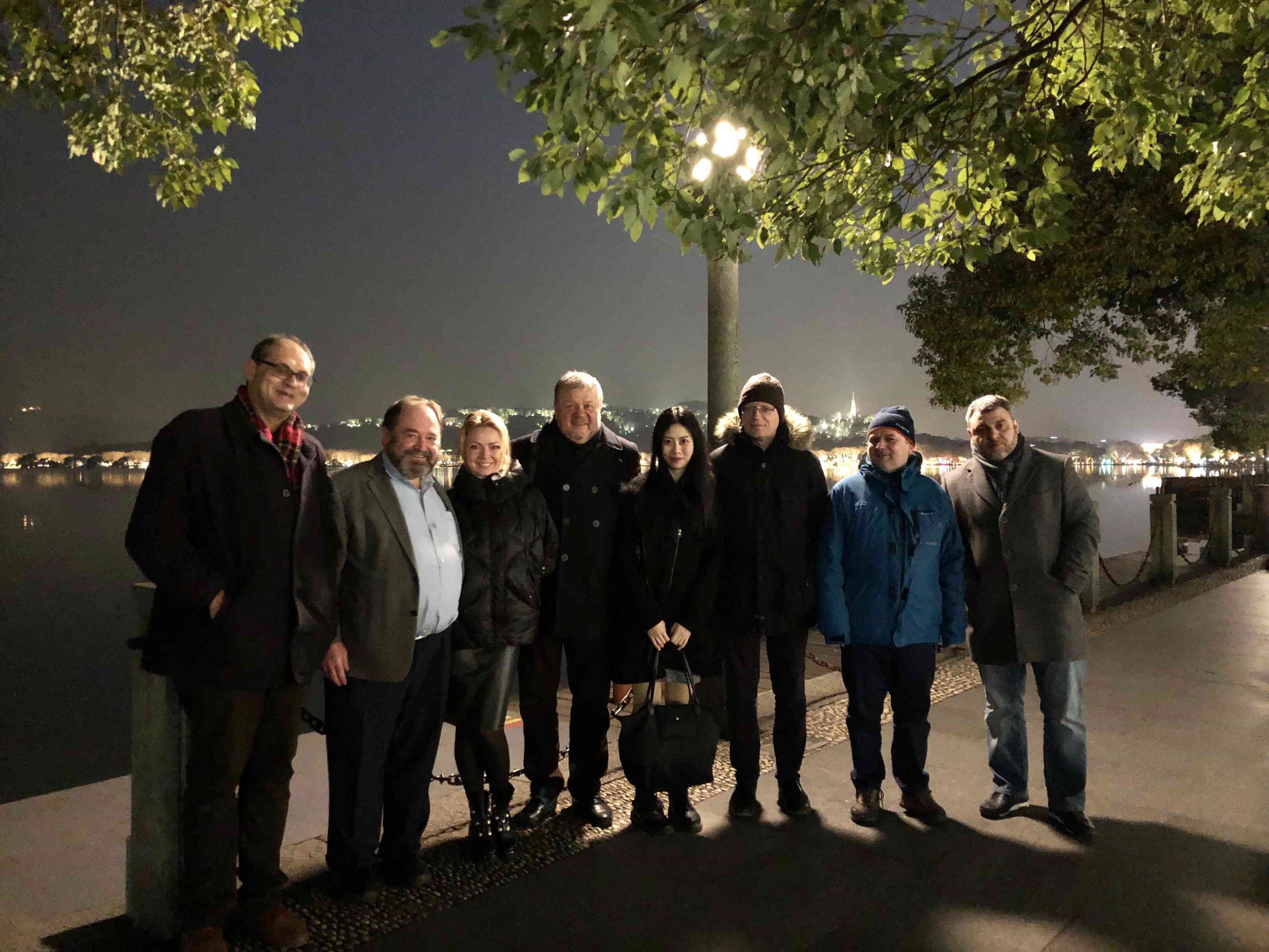
Fig. 1: Alexey Kavokin (third from the right) and Boris Altshuler (second from the left) and the team at Hangzhou’s West Lake.
The sparkle between two classical research fields
Research in one field may unexpectedly play an even more important role in another field, as it is often the case in science. And sometimes bringing two seemingly irrelevantphenomena togetherleads to remarkable findings. The Nernst effect in the Corbino disk is a good illustration to this rule.
To better explain this work, we’d better go back to 1911. An Italian physicist named Corbino attached two electrodes on the inner and outer edge of a disk and placed a magnetic field normal to the disk. The induced Lorentz force gives rise to magnetization currents (as shown in Fig. 2). Corbino ran the test with different metals and showed that the radial resistivity of the disk becomes stronger with the increase of the magnetic field. This is how the effect of magnetoresistance was found. This geometry setting is later named the "Corbino disk".
Over the years, scientist have studied the Hall effect in the Corbino geometry, in both classical and quantum limits. In contrast, the most important thermomagnetic effect, namely the Nernst effect, remains poorly explored in this disk geometry.
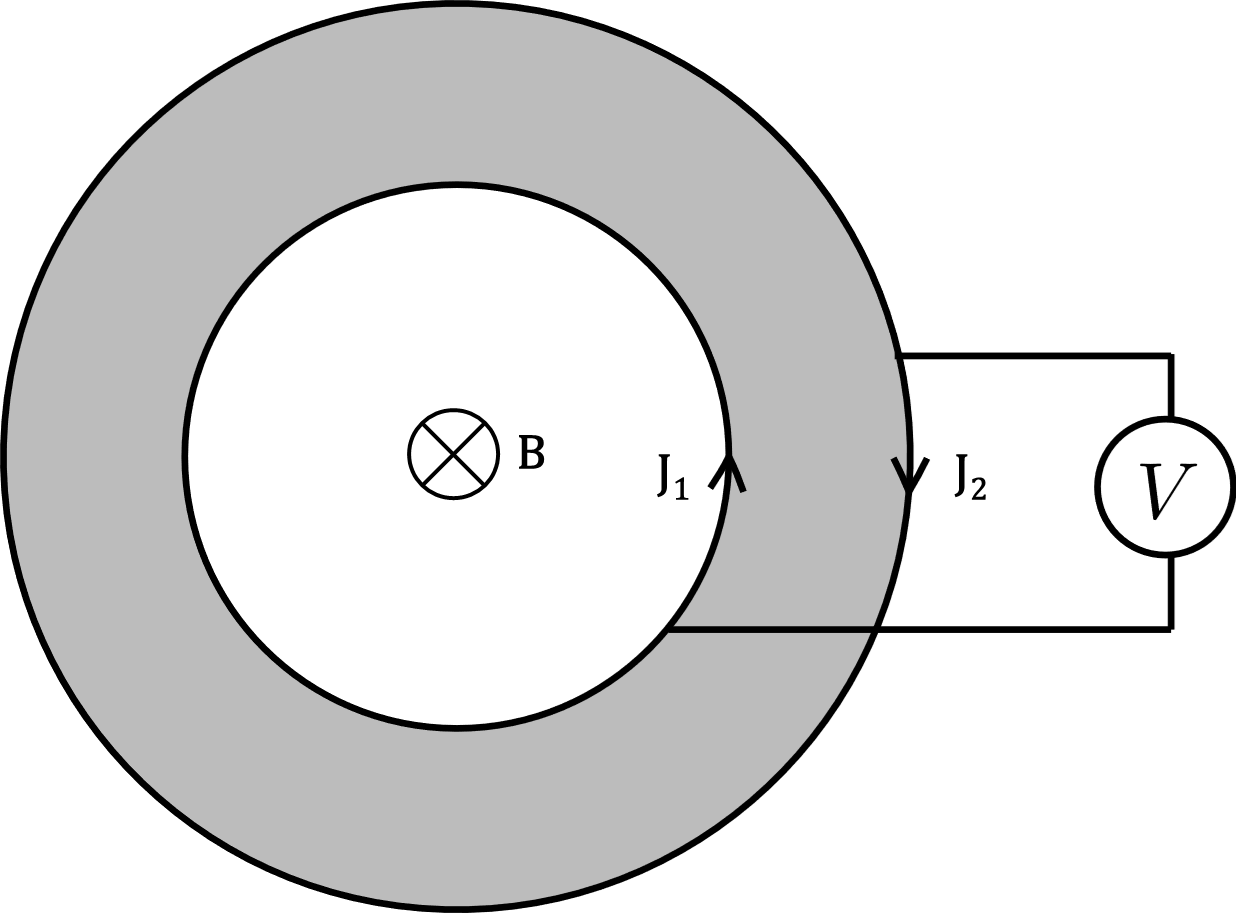
Fig. 2: A Corbino disk: In Corbino’s experiment, the inner and outer edges of the disk are connected to electrodes. A magnetic field perpendicular to the plane of the disk gives rise to magnetization currents.
The Nernst Effect is, as shown in Fig. 3, the induction of an electric current (in the y-direction) by an external magnetic field (in the z-direction) and a temperature gradient (in the x-direction). It was discovered in the 19th century by German scientist Walter Nernst and his supervisor Albert von Ettingshausen. Recently, the giant Nernst or Nernst–Ettingshausen effects have been observed in graphene, in a pseudogap phase of quasi–two-dimensional, high-temperature superconductors, and in conventional superconducting films being in the fluctuation regime.
How can we understand the Nernst effect? Generally speaking, the Nernst signal consists of two parts: a kinetic and a thermodynamic one. The former is governed by the conductivity of the sample and the derivative of the chemical potential of the carriers over temperature. The latter is related to the stationary magnetization currents induced by the temperature gradient. These currents are independent from the conductivity of the sample, which makes them unique and extremely interesting from a fundamental point of view. The research community started to gain some understandings of the thermodynamic part in 1964, whereas this problem has been readdressed in almost every decade due to its importance for the quantum Hall effect and superconductivity researches. However, so far, there hasn´t been any concrete experimental validation.

Fig. 3: The Nernst Effect: An electrical field (Ey) is induced by a magnetic field normal to the plane (Hz) and a temperature gradient in the x-direction (red arrow)
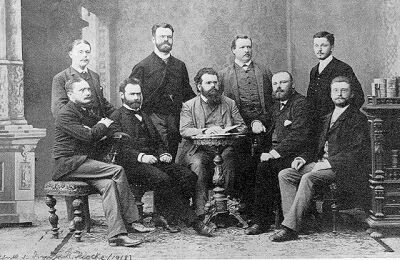
Fig. 4: Nobel laureate in Chemistry Walter Nernst (first from the left in the back) and his advisor Albert von Ettingshausen (second from the left in the front)
This time, Kavokin and his collaborators were onto some breakthrough.
The key to themuch awaitedvalidation is to prove the existence of magnetization currents. Magnetization currents are too elusive in ordinary geometries, e.g. rectangle bars, etc. That was when Kavokin and his colleagues looked into the Corbino disk.
"When there's a strong external magnetic field, the current does not propagate between the inner and outer edges of the disk, and therefore we can safely neglect the kinetic currents. In the presence of the magnetic field and temperature gradient, the Corbino disk would produce significant magnetization currents. We can therefore view the total currents in the sample as the magnetization currents," said Kavokin.
They calculated the magnetic field induced by the currents and found that the field experiences pronounced unharmonic oscillations. "We compared Corbino disks made of normal metal and of graphene and discovered that their oscillation behaviors are differ strongly different. The behavior predicted by our microscopic model can be experimentally measured by state-of-art technology, e.g. with a SQUID magnetometer in the center of the disk."
Towards new electronic devices and materials
Thanks to the Corbino disk, we finally have a chance to peek into the pure thermodynamic part of the Nernst effect. Kavokin said, "It has been a subject of debate for many years: the contribution of the diamagnetic (or magnetization) currents to the Nernst effect. Our work sheds light on it."
With the universal link established in this work, one could in turn extract information on carriers, which offer a powerful tool for the experimental studies of transport phenomena in 2D crystals. This is, by no doubt, great news for experimentalists. It may help developing new electronic devices and materials, and all together a brand-new electronic world.
PNAS is the official journal of the National Academy of Sciences of the United States of America. Founded in 1914, it is one of the most quoted multidisciplinary journals
Introduction to the author:
Professor Alexey Kavokin received his master’s degree in physics with honor from St-Petersburg State Technical University and his Ph.D. in Physical and Mathematical Sciences at A.F. Ioffe Institute, Russian Academy of Sciences in St Petersburg. He was the Chair of Nanoscience and Photonics and Professor of Physics and Astronomy School at University of Southampton. Prof. Kavokin accepted the offer as the Director of the International Center of Polaritonics and Chair Professor at Westlake University in June 2018. He has invited professors and researchers to join the center and work together on “quantum fluids of light”. He has also invited many international scholars to visit Westlake University for in-depth collaboration, including the co-author of the paper mentioned above, Boris Altshuler, who is a member of the American Academy of Arts and Sciences and Professor at Columbia University. Professor Kavokin establishing and managing a world-leading research center in Hangzhou, Zhejiang Province.
近日,《美国国家科学院院刊》(PNAS)发表题为The Nernst Effect in Corbino Geometry的研究论文。西湖大学讲席教授Alexey Kavokin为第一作者和通讯作者,哥伦比亚大学教授Boris Altshuler为共同通讯作者。
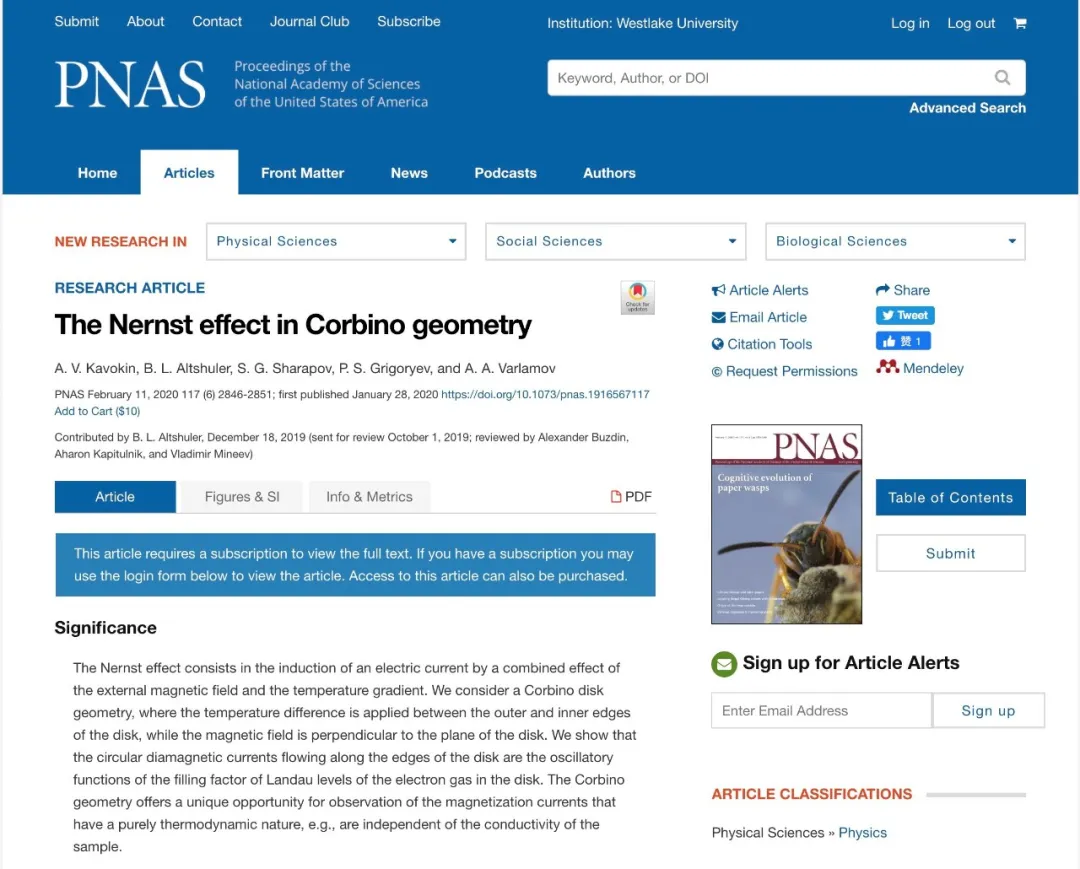
Kavokin和合作者研究发现,柯比诺圆盘这种独特的几何结构为观察能斯特效应中的热力学本源提供了一个非常理想的平台。根据理论计算,他们预言如果使得一个柯比诺圆盘的内外两侧存在温度差,则能在圆盘中心测得随外加垂直磁场而震荡变化的诱发磁场。
作者的灵感产生于哥伦比亚大学Boris Altshuler教授2019年访问西湖大学期间。短短三周的交流访问,让他们的思维碰出了火花,并完成了基本的理论推理。“接下去的几周,我们完成了所有的推导和计算,只用了纸和笔,这是非常美妙的一件事。”Kavokin说。
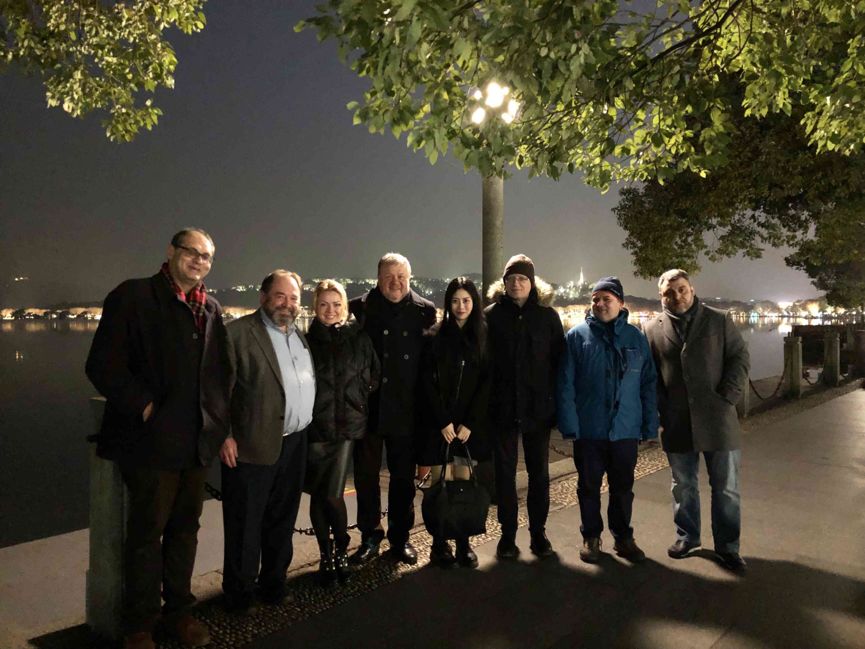
图1 :Alexey Kavokin(右三)、Boris Altshuler(左二)和团队夜游西湖
开云app官网下载安卓 就是这样,有的时候是“无心插柳”,即一个领域的研究,在另一个领域发挥了更大的作用;还有时,关联两个看起来毫无联系的作用现象,能带来惊人的发现。柯比诺圆盘里的能斯特效应,就属于后者。
要理解这项研究,我们首先要回到1911年。意大利物理学家柯比诺(Corbino)在一个导电圆盘的内外侧接上两个电极,在垂直圆盘方向加一个磁场;这个外加磁场引发的洛伦兹力产生磁化电流,该电流和圆盘的电导率无关。柯比诺利用不同金属做了一系列测试验证这一结论。后来这种圆盘被称为“柯比诺圆盘”。
人们利用柯比诺圆盘对霍尔效应做了很多深入研究,但用它对最重要的热磁效应——能斯特效应,做的研究却不多。
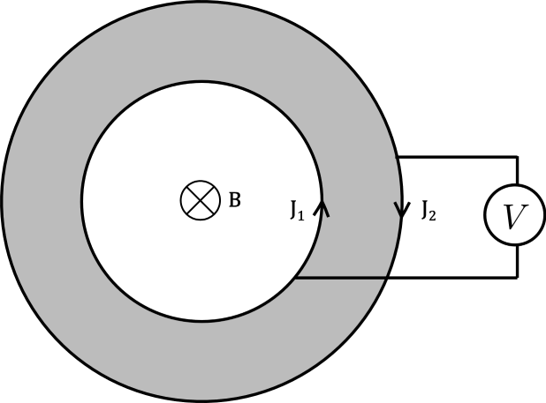
图2:柯比诺圆盘。在柯比诺的实验中,圆盘内外接电极并外加磁场会产生磁化电流。
再来看能斯特效应(图3)。在能斯特效应中,一个沿x方向的温度梯度和一个沿z方向的外加磁场共同作用可以导致一个沿y方向的感应电场。这是由德国科学家能斯特和他导师爱廷豪森(图4)在19世纪发现的。如今,科学家已陆续在石墨烯、准二维高温超导体的赝能隙相、常规超导薄膜中都观察到能斯特效应。
为什么会出现这样的效应?科学家们尝试解释说:能斯特效应中的感生电流由动力学(kinetic)和热力学(thermodynamic)两部分组成;前者与样品电导率、载流子化学势对温度的导数有关,后者只与温度梯度引起的磁化电流有关。对热力学部分的认识起始于1964年,之后由于它对量子霍尔效应和超导体系等研究的重要性而不断被重新讨论,但时至今日都没有确凿的实验证据。
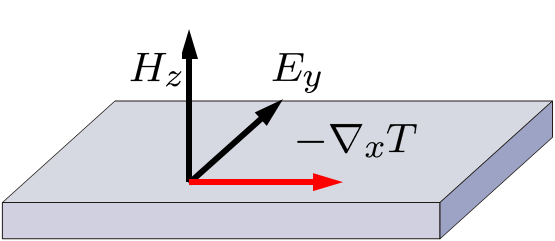
图3:能斯特效应。感生电场Ey,是垂直方向磁场Hz和沿x方向温度梯度(红箭头)共同作用的结果
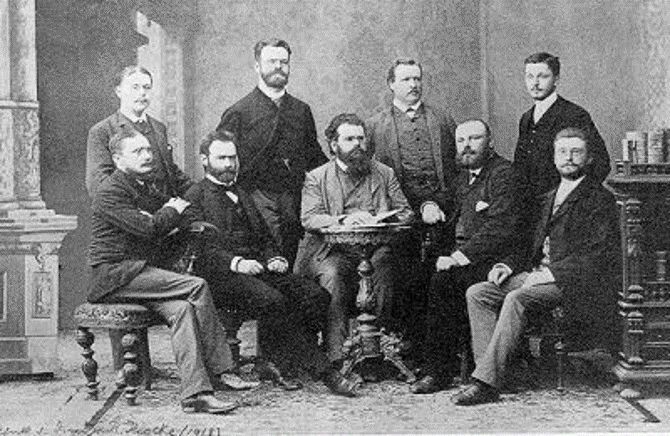
图4:1920年诺贝尔化学奖得主瓦尔特·能斯特(后排左一)和他导师阿尔伯特·爱廷豪森(前排左二)
这一次,Kavokin和他的合作者们取得了重要突破。
要检验对能斯特效应的解释正确与否,关键是要找到磁化电流。然而要测量到纯磁化电流,在通常的长方几何结构中根本不可能。Kavokin他们想到了用柯比诺圆盘。
“因为它的结构很特别,外加一个很强的磁场后,柯比诺圆盘中的电流不会在内外侧之间传播,因此我们可以忽略动力学电流,而此时的柯比诺圆盘在温度梯度下会出现明显的磁化电流,所以我们可以认为样品中的总电流基本就是磁化电流”,Kavokin说。
他们计算出由磁化电流感应生成的磁场,发现这个磁场会表现出非谐振震荡。“我们比较了一般金属和石墨烯材料,你会发现两者的震荡形式大不相同。我们通过建立模型所预测的磁场行为,以目前的技术已经可以开展实验验证,比如放一个SQUID磁力计到圆盘的中间。”
通过柯比诺圆盘,人们终于有机会窥探到能斯特效应中纯粹热力学的那部分。Kavokin说,“关于抗磁电流对能斯特效应的贡献,很多年来一直都存在争议。我们的工作为解决这个问题带来了希望。”
进一步借助这层关系,人们可以反过来推测样品载流子的信息,用在二维系统的输运研究中。这对实验人员来说,实在是个好消息!利用二维材料的输运性质,开发新的电子器件和电极材料,或许将构建起一个新的电子世界。
美国国家科学院院刊》(Proceedings of the National Academy of Sciences of the United States of America)是美国国家科学院的院刊,创刊于1914年。它是被引用次数最多的综合学科文献之一,也是世界公认的四大名刊(Cell,Nature,Science,PNAS)之一。
论文作者介绍
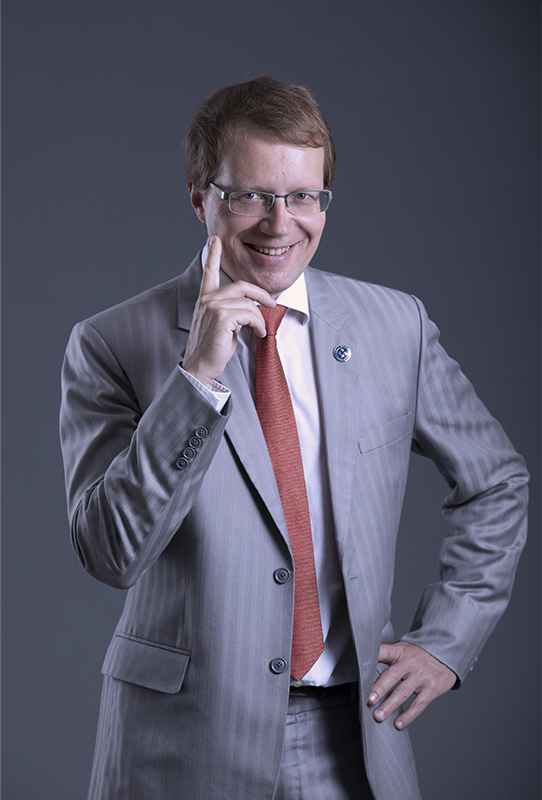
Kavokin教授,俄罗斯科学院物理技术研究所物理与数学科学博士,曾任南安普顿大学纳米物理和光子学主任、物理与天文学系讲席教授。2018年受聘为西湖大学理论物理讲席教授,创立了国际极化激元中心。他先后引进多位教授、研究员加入该中心,共同研究“液态光的量子”——极化激元;多次邀请国际知名专家来杭交流访问,开展深度合作研究,包括本项研究的合作者——美国科学院院士、哥伦比亚大学教授Boris Altshuler;为在浙江杭州建立一个国际领先的研究中心贡献了重要力量。